Autotrophic Nutrition: Harnessing Energy from the Sun
Have you ever wondered how a plant can grow so lush and green without consuming food as animals do? The secret lies in a fascinating process called autotrophic nutrition, which allows organisms to produce their own food using simple inorganic substances. This method is crucial for life on Earth, as it forms the foundation of most food chains. The ability of certain organisms to convert sunlight into energy is nothing short of miraculous. By understanding autotrophic nutrition, we gain insights into how life sustains itself and how energy flows through ecosystems.
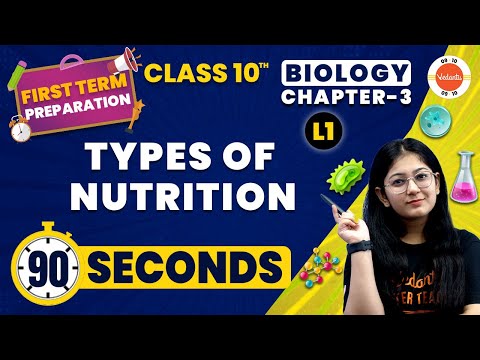
Photosynthesis: The Process of Converting Light to Energy
Photosynthesis is the cornerstone of autotrophic nutrition. This process occurs primarily in plants, algae, and certain bacteria, enabling them to convert light energy into chemical energy stored in glucose. Chlorophyll, the green pigment in plant cells, plays a vital role by capturing sunlight. During photosynthesis, carbon dioxide and water are transformed into glucose and oxygen, a reaction that sustains life on Earth. Without photosynthesis, the oxygen we breathe and the food we consume would not exist. This process not only fuels plant growth but also supports the energy needs of herbivores and, indirectly, carnivores.
Types of Autotrophs: Plants, Algae, and Cyanobacteria
Autotrophs are diverse, ranging from towering trees to microscopic algae. Plants are the most well-known autotrophs, using their leaves as solar panels to capture sunlight. Algae, found in aquatic environments, also perform photosynthesis, contributing significantly to global oxygen production. Cyanobacteria, often referred to as blue-green algae, are among the oldest life forms on Earth. These bacteria are crucial in many ecosystems, particularly in nutrient-poor environments where they can fix atmospheric nitrogen. The diversity of autotrophs highlights the adaptability of life and its ability to thrive in various conditions.
Heterotrophic Nutrition: Consuming Organic Matter
Unlike autotrophs, heterotrophs cannot produce their own food and must rely on consuming organic matter. This method of nutrition is prevalent among animals, fungi, and some bacteria. Heterotrophic organisms play a vital role in ecosystems by recycling nutrients and maintaining balance. By consuming other organisms, heterotrophs ensure the flow of energy through the food web. Understanding the various types of heterotrophic nutrition helps us appreciate the complexity and interconnectedness of life.
Herbivores: Plant-Based Diets
Herbivores are animals that primarily consume plants. They have evolved specialized digestive systems to break down tough plant materials, such as cellulose. Examples include cows, deer, and rabbits. These animals play a crucial role in controlling plant populations and serving as prey for carnivores. Their plant-based diet is essential for transferring energy from producers to higher trophic levels. Herbivores are often selective in their feeding habits, choosing specific plants or plant parts, which can influence plant community dynamics.
Carnivores: Meat-Based Diets
Carnivores are organisms that derive their energy and nutrients from consuming other animals. They have adaptations such as sharp teeth and claws to capture and kill prey. Examples include lions, eagles, and sharks. Carnivores help regulate prey populations, preventing overgrazing and promoting biodiversity. Their role in ecosystems is critical for maintaining ecological balance. Some carnivores are apex predators, sitting at the top of the food chain, while others may be secondary or tertiary consumers.
Omnivores: A Mixed Diet Approach
Omnivores have the ability to consume both plant and animal matter, giving them a versatile diet. Humans, bears, and pigs are examples of omnivores. This dietary flexibility allows them to adapt to various environments and food availability. Omnivores contribute to ecosystem stability by occupying multiple trophic levels. Their varied diet can influence their role in the food web, as they may act as both predators and prey, depending on the context.
Saprotrophic Nutrition: Decomposers in Action
Saprotrophic nutrition involves the breakdown of dead organic matter by decomposers. This process is essential for nutrient recycling and soil fertility. Fungi and bacteria are the primary saprotrophs, breaking down complex organic compounds into simpler substances. Without saprotrophs, ecosystems would be overwhelmed with dead material, and nutrient cycling would cease. By decomposing organic matter, these organisms release nutrients back into the soil, making them available for plant uptake and supporting new growth.
Role of Fungi and Bacteria in Decomposition
Fungi and bacteria are the unsung heroes of decomposition. Fungi, with their extensive network of hyphae, penetrate organic matter, secreting enzymes that break down complex compounds. Bacteria, on the other hand, thrive in various environments, decomposing organic material at a microscopic level. Their combined efforts ensure the efficient recycling of nutrients. Decomposers also play a role in carbon cycling, as they release carbon dioxide back into the atmosphere during the breakdown of organic matter.
Importance of Saprotrophs in Ecosystems
The presence of saprotrophs is crucial for ecosystem health. They prevent the accumulation of dead material, which can harbor pathogens and pests. By recycling nutrients, saprotrophs support plant growth and productivity. Their activity influences soil structure and fertility, impacting plant community composition. In aquatic environments, saprotrophs contribute to the breakdown of organic matter, maintaining water quality and supporting aquatic life.
Parasitic Nutrition: Living Off a Host
Parasitic nutrition involves one organism, the parasite, deriving nutrients from another living organism, the host. This relationship can be detrimental to the host, as parasites often cause harm or disease. However, parasites play a role in controlling host populations and driving evolutionary adaptations. Their interactions with hosts are complex and can influence entire ecosystems. Understanding parasitic nutrition sheds light on the dynamics of host-parasite relationships and their impact on biodiversity.
Endoparasites vs. Ectoparasites: Internal and External Feeding
Endoparasites live inside the host’s body, feeding on tissues or absorbing nutrients from the host’s digestive system. Examples include tapeworms and flukes. Ectoparasites, on the other hand, live on the host’s surface, feeding on blood or other secretions. Fleas, ticks, and lice are common ectoparasites. Both types of parasites have evolved specialized adaptations to exploit their hosts effectively. Their presence can lead to various health issues in hosts, influencing population dynamics and species interactions.
Impact of Parasitism on Host Organisms
Parasitism can have profound effects on host organisms, ranging from mild discomfort to severe disease and death. The energy and resources diverted to combat parasites can affect host growth, reproduction, and survival. In some cases, parasitism can lead to coevolution, where hosts and parasites develop adaptations in response to each other. The impact of parasitism extends beyond individual hosts, influencing population dynamics, community structure, and ecosystem processes.
Symbiotic Nutrition: Mutual Benefits in Nature
Symbiotic nutrition involves close interactions between different species, where at least one benefits. These relationships can be mutualistic, commensal, or parasitic. Symbiosis is a driving force in evolution, leading to diverse adaptations and ecological niches. By forming partnerships, organisms can access resources and habitats that would otherwise be unavailable. Symbiotic relationships are found across all ecosystems, highlighting the interconnectedness of life.
Mutualism: A Win-Win Relationship
Mutualism is a type of symbiotic relationship where both species benefit. Examples include pollinators and flowering plants, where pollinators receive nectar, and plants achieve fertilization. Another example is the relationship between nitrogen-fixing bacteria and legumes, where bacteria provide nitrogen, and plants offer carbohydrates. Mutualistic interactions enhance survival and reproductive success for both partners. These relationships can influence species distributions, community dynamics, and ecosystem functioning.
Commensalism: One Benefits, the Other Unharmed
In commensalism, one species benefits while the other remains unaffected. An example is barnacles attaching to a whale, where barnacles gain mobility and access to food, while the whale is neither harmed nor helped. Commensal relationships can provide insights into the complexity of ecological interactions. These interactions may influence species distributions and community structure, as they can affect resource availability and habitat use.
Chemoautotrophic Nutrition: Energy from Chemical Reactions
In the absence of sunlight, some organisms have evolved to harness energy through chemical reactions. This process, known as chemoautotrophic nutrition, is vital in environments where light is scarce. These organisms, primarily bacteria and archaea, oxidize inorganic molecules such as hydrogen sulfide, ammonia, or iron to obtain energy. This unique method of nutrition allows life to thrive in extreme environments like hydrothermal vents and sulfur-rich hot springs. By converting chemical energy into organic compounds, chemoautotrophs form the base of the food chain in these isolated ecosystems.
Deep-Sea Vents: Life Without Sunlight
Deep-sea hydrothermal vents are among the most extreme environments on Earth, yet they host diverse communities of organisms. Here, chemoautotrophic bacteria form the foundation of the ecosystem. These bacteria utilize the chemical energy released from vent minerals to produce organic matter. Their presence supports a variety of life forms, including giant tube worms, clams, and shrimp, which rely on these bacteria for sustenance. The discovery of these ecosystems revolutionized our understanding of life’s adaptability and the potential for life beyond Earth.
Role of Chemosynthesis in Extreme Environments
In environments where sunlight cannot penetrate, chemosynthesis provides an alternative pathway for life. This process is not limited to deep-sea vents but is also found in sulfur-rich caves and cold seeps. Organisms in these habitats have developed unique adaptations to exploit chemical energy sources. Chemosynthesis highlights the remarkable resilience of life and its ability to colonize seemingly inhospitable environments. The study of these systems offers insights into the potential for life in extraterrestrial settings, such as the subsurface oceans of icy moons.
Mixotrophic Nutrition: Combining Multiple Methods
Mixotrophic nutrition represents a flexible approach to obtaining energy and nutrients, combining aspects of autotrophic and heterotrophic methods. This strategy is employed by various organisms, including some protists and plants. Mixotrophy allows organisms to adapt to changing environmental conditions and resource availability. By utilizing multiple nutritional pathways, mixotrophs can optimize their energy intake and enhance survival in dynamic ecosystems.
Examples of Mixotrophic Organisms
Mixotrophy is observed in a range of organisms, from single-celled protists to complex plants. For instance, certain algae can photosynthesize and ingest organic particles, while some carnivorous plants, like the Venus flytrap, can photosynthesize and capture insects for additional nutrients. These organisms exemplify the versatility of mixotrophy and its role in ecological interactions. By balancing autotrophic and heterotrophic nutrition, mixotrophs can exploit diverse habitats and resources.
Advantages of Mixotrophy in Changing Environments
The ability to switch between nutritional modes provides mixotrophs with a competitive edge in fluctuating environments. For example, in nutrient-poor waters, mixotrophic algae can supplement their diet by ingesting bacteria or organic matter. This adaptability enhances their resilience to environmental stressors, such as nutrient depletion or light limitation. Mixotrophy also influences community dynamics, as these organisms can occupy multiple ecological niches, affecting energy flow and nutrient cycling.
Human Nutrition: A Complex Interplay of Methods
Human nutrition is a multifaceted process that involves the intake and utilization of various nutrients to support growth, maintenance, and health. It encompasses a balance of macronutrients and micronutrients, each playing a crucial role in bodily functions. Understanding human nutrition is essential for promoting health and preventing disease. By exploring the complexities of our dietary needs, we can make informed choices that enhance well-being and longevity.
Macronutrients and Micronutrients: Essential Components
Macronutrients, including carbohydrates, proteins, and fats, provide the energy and building blocks necessary for bodily functions. Carbohydrates are the primary energy source, while proteins support tissue repair and enzyme production. Fats are vital for energy storage and cell membrane integrity. Micronutrients, such as vitamins and minerals, are required in smaller amounts but are equally important for health. They play roles in immune function, bone health, and metabolic processes. A balanced diet ensures adequate intake of both macro and micronutrients, supporting overall health.
The Role of Diet in Human Health and Disease Prevention
Diet plays a pivotal role in maintaining health and preventing chronic diseases. Nutrient-rich foods support immune function, reduce inflammation, and promote healthy aging. Conversely, poor dietary choices can contribute to obesity, cardiovascular disease, and diabetes. Adopting a balanced diet rich in fruits, vegetables, whole grains, and lean proteins can mitigate disease risk and enhance quality of life. Public health initiatives often focus on promoting healthy eating patterns to combat the rising prevalence of diet-related illnesses.
Conclusion
The diverse methods of nutrition in biology illustrate the complexity and adaptability of life. From autotrophic processes that harness sunlight to heterotrophic consumption of organic matter, each method plays a vital role in sustaining ecosystems. Understanding these nutritional strategies enhances our appreciation of life’s interconnectedness and resilience. As we continue to explore the intricacies of nutrition, we gain insights into the mechanisms that drive biological diversity and ecological balance.
Exploring the Diverse Methods of Nutrition in Biology
What are the primary methods of nutrition in biology?
In biology, the primary methods of nutrition include autotrophic and heterotrophic nutrition. Autotrophs, like plants, produce their own food through photosynthesis or chemosynthesis, while heterotrophs, such as animals, obtain nutrition by consuming other organisms.
How does autotrophic nutrition differ from heterotrophic nutrition?
Autotrophic nutrition involves organisms producing their own food using light or chemical energy, commonly seen in plants and some bacteria. In contrast, heterotrophic nutrition requires organisms to ingest or absorb organic substances, as seen in animals and fungi.
What role do decomposers play in the nutrition cycle?
Decomposers, such as fungi and bacteria, play a crucial role in the nutrition cycle by breaking down dead organic matter, recycling nutrients back into the ecosystem, and supporting the growth of autotrophic organisms.
Can you explain the process of photosynthesis in autotrophic nutrition?
Photosynthesis is a process used by plants and some bacteria to convert light energy into chemical energy stored in glucose. It involves the absorption of sunlight by chlorophyll, carbon dioxide intake, and water, resulting in the production of oxygen and glucose.
What is chemosynthesis and where does it occur?
Chemosynthesis is a process by which certain organisms, such as bacteria found in hydrothermal vents, produce food using chemical energy derived from reactions involving inorganic molecules, rather than sunlight.
How do carnivorous plants obtain their nutrition?
Carnivorous plants, like the Venus flytrap, obtain nutrition by trapping and digesting insects and other small animals. They supplement their nutrient intake from the soil with the nutrients obtained from their prey.
What is the significance of symbiotic relationships in nutrition?
Symbiotic relationships, such as those between legumes and nitrogen-fixing bacteria, are significant in nutrition as they enhance nutrient availability and absorption, benefiting both organisms involved and contributing to ecological balance.